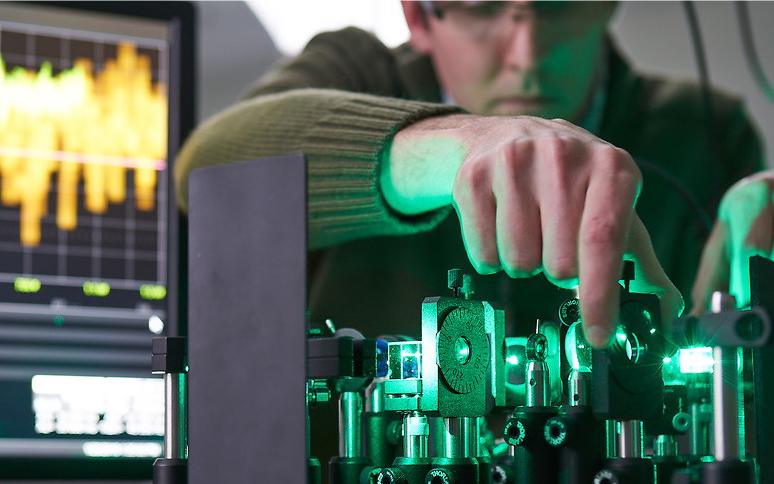
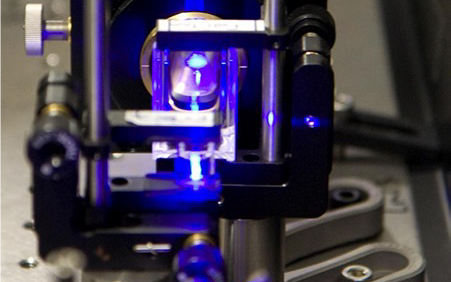
Rydberg Atom-Based Sensor
Rydberg atom-based radio frequency sensors work by tailoring the atomic response to a probe laser using other laser fields. The atoms are room temperature and contained in a vapour cell, a body that ordinarily contains gaseous cesium for radio frequency sensing.
The typical, and original, approach to this type of sensor is shown in Fig. 1. A probe and coupling laser form an electromagnetically induced transparency system that couples the D2 transition of cesium to a Rydberg state. When a radio frequency field is present that is near resonant with a transition from the Rydberg state, the transmission of the probe laser passing through the vapour in the vapour cell is changed. The changes in transmission can be used to determine the properties of the radio frequency field.
Advantages of the method are that the sensor is self-calibrated and traceable; the sensor can be all dielectric, hence highly electromagnetically transparent; the same sensor can have a high carrier bandwidth spanning from sub-GHz to THz; the sensor can be made in almost any shape and size; and the atoms can be very sensitive. We are pursuing several projects that address applications for Rydberg atom-based sensors as well as improvements in their functionality and manufacturability.
b) The experimental setup for testing. The antenna shown is the antenna under test. Note that the entire drawing consists of parts that can be dielectric like the vapour cell and waveguides to carry the light to the vapour cell. The photodetector can be located far from the electromagnetic field probe.
Representative Publications:
- “Microwave electrometry with Rydberg atoms in a vapour cell using bright atomic resonances,” J. Sedlacek, A. Schwettmann, H. Kubler, R. Low, T. Pfau, and J.P. Shaffer, Nature Physics 8,819 (2012).
- “Rydberg atom-based electric field sensing from radio to terahertz frequencies,” H.Q. Fan, S. Kumar, H. Kubler, S. Karimkashi and J.P. Shaffer, Journal of Physics B 48, 202001 (2015).
- “A read-out enhancement for microwave electric field sensing with Rydberg atoms,” J. P. Shaffer and H. Kübler, Proc. SPIE 10674, Quantum Technologies (2018).
Vapour Cell Atomic Clocks
Modern optical clocks are the most precise instruments that humanity has built. Highly stable time references play an important role in applications like radar, communications and navigation. Optical clocks are receiving increasing attention because they have outperformed microwave frequency references in the important metrics of stability and systematic uncertainty. The development of stable frequency combs for converting the optical frequency to a radio frequency timing reference has accelerated interest in optical clocks.
Unfortunately, laboratory optical clocks, like the strontium lattice clock, are not projected to be portable any time soon. Many important applications, e.g., radar, communications, and navigation, require fieldable devices with volumes of ~30 liters (or less) and fractional frequency instabilities that are ≤10-14 / √T. Compact clocks with low frequency instability are required. Vapour cell-based optical clocks have a realistic potential for achieving these metrics.
We are currently leveraging our expertise in high resolution spectroscopy and vapour cell engineering to develop portable optical atomic clocks, predominantly for timing applications that are required for data fusion, especially for radar.
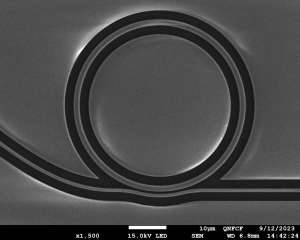
Interested in Collaborating or Joining Our Team?
You can find some of our work described in more detail by investigating the following project descriptions. If you are interested in collaborating with us or becoming a technical staff member, including student internships and postdoctoral training, please contact:
James P. Shaffer
Senior Technical Fellow
Quantum Valley Ideas Lab
(226) 791-4709
jshaffer@qvil.ca